Research Group “Applied Molecular Biology”
The aim of the ERC Advanced Investigator Group of Univ.-Prof. Dr. Dr. h. c. Werner E.G. Müller, which includes the groups of Prof. Dr. Xiaohong Wang, Prof. Dr. Dr. Heinz C. Schröder, Dr. Matthias Wiens and Dr. Meik Neufurth, is to gain new knowledge in basic research that can be transferred to medical applications.From biomolecules to biominerals: evolution of medically relevant metabolic pathways
The starting point of our current research is our long-term investigation of secondary metabolites of sponges, one of the richest sources of new, potentially medically applicable bioactive compounds in the sea. Among others, we succeeded in finding an inhibitor of the Herpes simplex virus DNA polymerase and elucidating its mechanism: araA, a virostatic that is still in clinical use today. The mechanism of action of another nucleoside analogue, araC, which is used in tumor therapy, was also clarified. In the course of this work, we discovered and investigated in sponges metabolic pathways and mechanisms, such as apoptosis. This led us also to understand the molecular basis of cell death in human diseases such as Alzheimer's disease, HIV and Creutzfeldt-Jakob disease, as well as the protective effect of certain drugs, such as Memantine and Flupirtine. It became increasingly clear that the basic metabolic processes and stress response mechanisms in sponges are similar to those in humans. This ultimately led to the discovery that all animals are of monophyletic origin.Biomineralization - an enzyme-catalyzed process?
A fundamental question was: How do skeletal organisms overcome the activation energy barriers that also apply to mineralization processes? We know from biochemistry that enzymes can reduce the activation energy of chemical reactions. Therefore we asked: Is also the formation of the silica skeleton (glass skeleton) of the siliceous sponges enzyme-catalyzed? In fact, we could demonstrate this. As we have been able to show, the sponge protein silicatein has the ability to synthesize silica (i.e. an inorganic material) from orthosilicate (also a purely inorganic material) via an enzymatic mechanism. Until then, no other enzyme had been able to perform such a reaction – a discovery that was examined in detail in an ERC Advanced Grant project ("BIOSILICA") by the head of the research group, not only for technical (silica is a basic material in nanotechnology), but also of great importance for medical applications.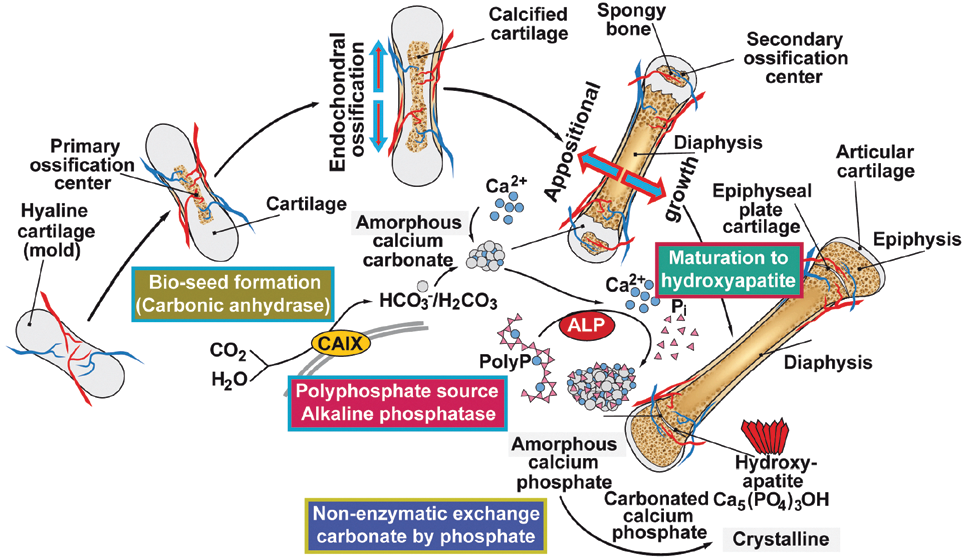
Next we asked: Is also the formation of calcium carbonate and calcium phosphate skeletons enzymatically catalyzed? We found that during bone mineralization, hydroxyapatite is not initially formed, but amorphous calcium carbonate (ACC), synthesized by a carbonic anhydrase. The ACC is then converted to amorphous calcium phosphate (ACP) by carbonate-phosphate exchange, and finally hydroxyapatite. The required phosphate is supplied – again enzymatically – by the hydrolysis of inorganic polyphosphates (polyP), catalyzed by the alkaline phosphatase (ALP), a key enzyme of the osteoblasts.
Tissue regeneration: who supplies the energy extracellularly?
An extracellular energy source was unknown – ATP, the universal energy carrier, is only released from cells in minimal amounts. We therefore asked: Where does this energy come from, who supplies it? The answer is: polyP. These polymers, which consist of numerous phosphate residues linked together via high-energy phosphoanhydride bonds, are not only a phosphate source for bone mineralization, but, as we found, generally serve as a storage and donor of metabolic energy, especially in the extracellular space. During their hydrolysis, a multiple of the free energy of the ATP hydrolysis is released. We were able to show that the energy stored in polyP (a multiple of the energy released during the hydrolysis of ATP) can be converted extracellularly into biochemically usable energy, in the form of ATP, through the combined action of two enzymes, the cell membrane-bound ALP and the adenylate kinase (ADK).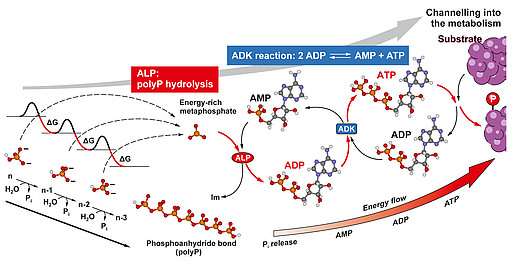
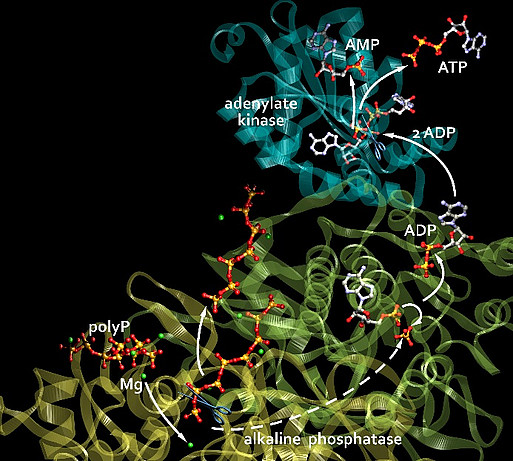
Development of medical applications
The elucidation of these unusual properties of the amorphous inorganic biomaterials ACC, ACP, silica and polyP (as nano/microparticles) enabled us to develop new strategies for tissue engineering and applications in regenerative medicine. These materials are not only regeneratively active and biodegradable, but also serve, in the case of polyP, as a source of metabolic energy – a property combination that has not yet been shown by any other material for tissue engineering/tissue repair. By applying a bio-inspired procedure and using different divalent metal ions (counterions) we succeeded in fabricating amorphous (non-crystalline, i.e. biologically active) nano- and microparticles of polyP with different biological properties. These nano/microparticles are stable and become biologically active after conversion into a coacervate in the presence of protein-containing body fluids (e.g. wound secretions): for example, Ca-polyP nano/microparticles, after coacervate conversion, show a strong stimulating effect on bone mineralization.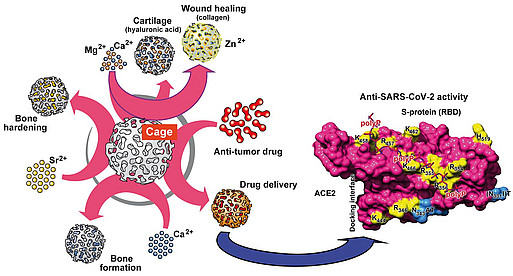
By combining polyP or polyP nano/microparticles with hydrogel-forming polymers such as alginate, hyaluronic acid, chitosan derivatives or chondroitin sulfate, we were able to develop hybrid materials (even 3D-printable) for a number of medical applications, from tooth sealing to wound healing materials, to stimulate microvascularization and bone and cartilage regeneration, as well as to manufacturing of artificial blood vessels and corneas. With suitable hydrogels, it was also possible to develop a polyP-based bio-ink for 3D cell printing (3D bioplotting) – the embedded cells remained proliferatively active. The first regeneratively active implant materials have already been tested in animal experiments and are to be brought into clinical use.
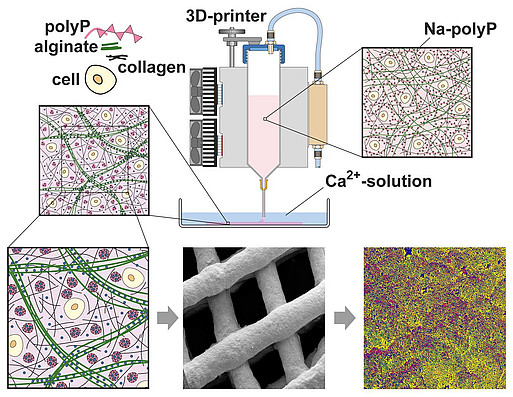
In addition to this work funded by three ERC proof-of-concept grants ("Si-Bone-PoC", "MorphoVES-PoC" and "ArthroDUR") and several other EU projects coordinated by us, we are currently developing, in a German-Chinese project, novel wound healing materials, especially for chronic wounds. Like other regenerative processes, wound healing requires a lot of energy – a need that is met by the polyP component of these materials. In addition, polyP induces microvascularization. In cooperation with the Department of Dermatology of the University Medical Center, it was already shown that a wound pad based on polyP can be successfully applied to difficult-to-heal chronic wounds in patients. Further research in the EU Horizon 2020 project "InnovaConcrete" uses the stabilizing properties of polyP on ACC to give cements (both technical and medically applicable) self-healing properties.
Antiviral compounds against SARS-CoV-2
Polyphosphates are not only morphogenetically active, they also have antiviral properties. We were able to show this earlier in HIV-1 infected cells. Now we have been able to show that, in model systems, polyP has a protective effect against the coronavirus SARS-CoV-2. PolyP binds to the receptor binding domain of the spike proteins of SARS-CoV-2 and thereby blocks their attachment to the host cell receptor protein ACE2. And not only that: PolyP induces the expression of mucin proteins which build the protective mucus layer that viruses have to cross in order to reach the cells of the nasopharynx epithelium.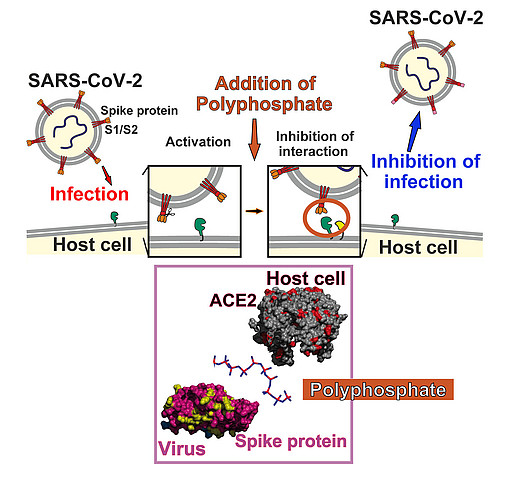